Phylogenetic Tree In Bioinformatics: In the field of bioinformatics, the phylogenetic tree is used to study the evolutionary relationships between species. By analyzing genetic, morphological, or molecular data, scientists can trace the lineage of organisms, identifying how they evolved from common ancestors over time. These trees serve as a blueprint of life’s evolutionary journey, helping researchers understand biodiversity, species classification, and genetic variations.
But why are phylogenetic trees so important in bioinformatics, and how do they work? This blog explores the importance, types, significance, and applications in scientific research and clinical studies.
What is a Phylogenetic Tree?
A phylogenetic tree is a branching diagram that represents the evolutionary relationships among different biological species based on similarities and differences in their physical or genetic characteristics.
Essentially, it traces the lineage of species, showcasing how they have diverged from common ancestors over time.
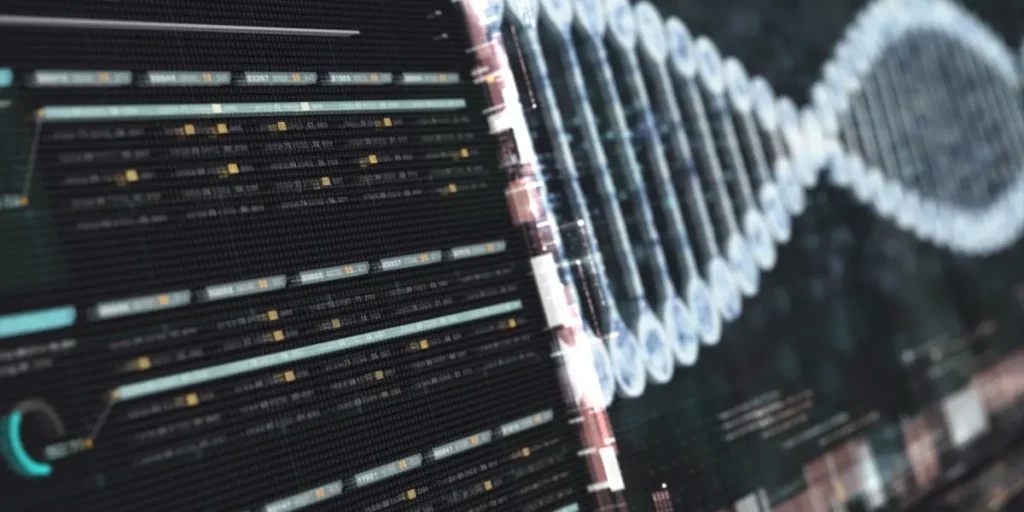
Importance of Phylogenetic Trees in Bioinformatics
In bioinformatics, phylogenetic trees are indispensable for several reasons:
- To understand evolutionary relationships: They help scientists decipher the evolutionary pathways of organisms, providing insights into how species have evolved and diversified.
- Classification of organisms: By analyzing genetic data, phylogenetic trees assist in the accurate classification of organisms, ensuring a systematic arrangement based on evolutionary lineage.
- Predicting function of genes and proteins: By examining the position of genes within a phylogenetic tree, researchers can predict the function of unknown genes based on their proximity to known genes.
- Tracing the spread of diseases: Phylogenetic analysis is crucial in understanding the transmission patterns of pathogens, aiding in the control and prevention of diseases.
Types of Phylogenetic Trees
Phylogenetic trees can be categorized based on their structure and the information they convey:
- Rooted Trees: These trees have a single ancestral lineage (root) from which all other nodes descend, indicating a common ancestor.
- Unrooted Trees: Unlike rooted trees, unrooted trees depict relationships among species without specifying a common ancestor, focusing on the relatedness among various nodes.
- Bifurcating Trees: Each internal node gives rise to exactly two descendant nodes, representing a dichotomous split.
- Multifurcating Trees: Here, an internal node may lead to multiple descendants, indicating simultaneous divergence events.
- Cladograms: These trees represent only the branching order without indicating branch lengths. They are used primarily to show relative relationships among species.
- Phylograms: These trees include branch lengths that represent evolutionary distances. The length of the branches indicates the amount of evolutionary change.
- Dendrograms: A general term for trees that depict hierarchical relationships, dendrograms can be used in various contexts, including phylogenetic studies and clustering analyses.
Parts of a Phylogenetic Tree
The parts of a phylogenetic tree are branches, nodes, leaves, and the root.
- Nodes: Points where a lineage splits, representing common ancestors.
- Branches: Lines connecting nodes, indicating evolutionary pathways.
- Root: The common ancestor of all entities in the tree.
- Leaves (Tips): The end points of the tree, representing current species or sequences.
Significance of Phylogenetic Trees
The significance of phylogenetic trees extends beyond mere representation of evolutionary relationships:
- Conservation biology: They guide conservation efforts by identifying species that are evolutionarily unique and may require prioritized protection.
- Comparative genomics: By comparing genomes across species, phylogenetic trees help identify conserved elements and understand genomic evolution.
- Drug discovery: Understanding the evolutionary relationships of pathogens can inform the development of effective treatments.
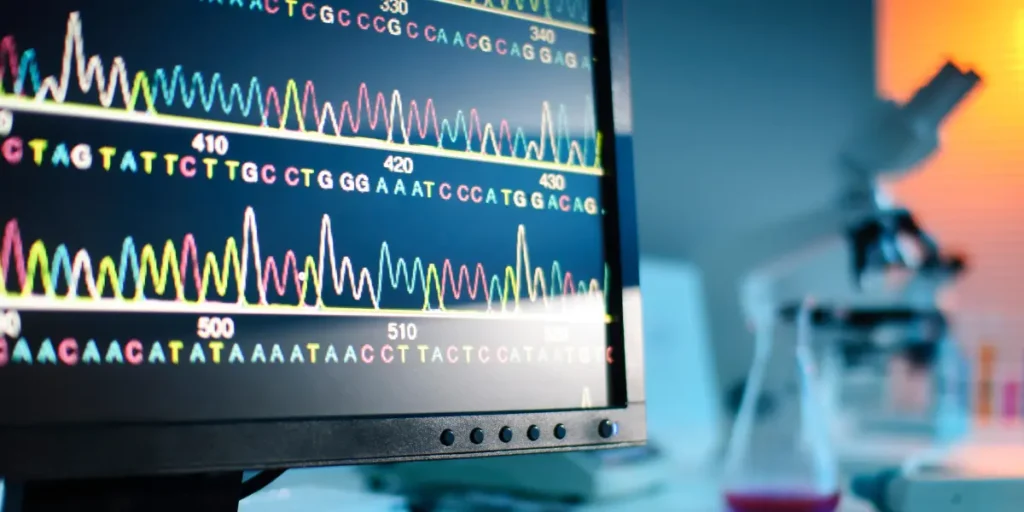
How to Analyze a Phylogenetic Tree
Analyzing a phylogenetic tree involves several steps:
- Data collection: Gather genetic sequences of the species under study.
- Sequence alignment: Align the sequences to identify regions of similarity.
- Tree construction: Use computational methods to construct the tree based on the aligned sequences.
- Interpretation: Examine the tree to infer evolutionary relationships and draw conclusions.
How Phylogenetic Trees Work
Phylogenetic trees work by analyzing genetic or morphological data to reconstruct the evolutionary history of species. By comparing sequences, scientists can infer the order of divergence and the relatedness among species.
For those keen on delving deeper into bioinformatics and clinical research, the Learning Labb Research Institute (LLRI) offers courses tailored to equip learners with essential skills. Their programs encompass clinical research, bioinformatics, and more, providing a robust foundation for aspiring professionals.
On A Final Note…
The phylogenetic tree in bioinformatics is more than just a diagram; it’s a window into the evolutionary past, offering insights that are crucial for various scientific endeavors. By understanding its importance, types, and significance, we can better appreciate the intricate tapestry of life on Earth.
“The tree of life is a powerful image that conveys the idea of evolution and the interconnectedness of all living things.” – Charles Darwin